To handle the "little work" suggested by Yves in the comments, geometry suggests a rigorous and fully general proof.
If you like, you may replace all references to areas by integrals and references to "arbitrary" by the usual epsilon-delta arguments. The translation is easy.
To set up the picture, let $G$ be the survival function
$$G(x) = 1-F(x) = \Pr(X \gt x).$$
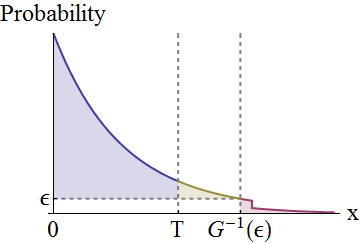
The figure plots a part of $G$. (Notice the jump in the graph: this particular distribution is not continuous.) A large threshold $T$ is shown and a tiny probability $\epsilon \le G(T)$ has been selected (so that $G^{-1}(\epsilon)\ge T$).
We're ready to go: the value we're interested in, $\epsilon F^{-1}(1-\epsilon) = \epsilon G^{-1}(\epsilon)$ (the one we want to show converges to zero), is the area of the white rectangle with height $\epsilon$ and base from $x=0$ to $x= G^{-1}(\epsilon)$. Let's relate this area to the expectation of $F$, because the only assumption available to us is that this expectation exists and is finite.
The positive part $E_{+}$ of the expectation $\mathbb{E}_F(X)$ is the area under the survival curve (from $0$ to $\infty$):
$$\mathbb{E}_F(X) = E_{+}-E_{-} = \int_0^\infty G(x) dx - \int_{-\infty}^0 F(x) dx.$$
Because $E_{+}$ must be finite (for otherwise the expectation itself would not exist and be finite), we may pick $T$ so large that the area under $G$ between $0$ and $T$ accounts for all, or nearly all, of $E_{+}$.
All the pieces are now in place: the graph of $G$, the threshold $T$, the small height $\epsilon$, and the right-hand endpoint $G^{-1}(\epsilon)$ suggest a dissection of $E_{+}$ into areas we can analyze:
As $\epsilon$ goes to zero from above, the area of the white rectangle with base $0\le x \lt T$ shrinks to zero, because $T$ remains constant. (This is why $T$ was introduced; it's the key idea to this demonstration.)
The blue area can be made as close to $E_{+}$ as you might like, by starting with a suitably large $T$ and then choosing small $\epsilon$.
Consequently, the area left over--which clearly is no greater than the white rectangle with base from $x=T$ to $x=G^{-1}(\epsilon)$--can be made arbitrarily small. (In other words, just ignore the red and gold areas.)
We have thereby broken $\epsilon G^{-1}(\epsilon)$ into two pieces whose areas both converge to zero. Thus, $\epsilon G^{-1}(\epsilon)\to 0$, QED.